
In the last decade, genome editing in biomedical laboratories across the world has become commonplace and the use of animals in the early stages of this research has led to ground-breaking discoveries to treat disease.

Lluis Montoliu, a research scientist at CNB-CSIC & CIBERER-ISCIII, Madrid, Spain, looks at some of the most successful studies and sees a future where this technology could be used to prevent disease, not just treat it.
Ten years ago, the seminal publication** by future Nobel Prize winners, Emmanuelle Charpentier and Jennifer Doudna, opened up opportunities to apply genome editing tools, and particularly CRISPR-Cas9 technology, to investigate the genetic basis of many diseases in animals, including humans.

While there are other gene-editing technologies, CRISPR (also commonly called ‘genetic scissors’) has revolutionised our ability to introduce specific genetic changes into experimental animal models, both more precisely and faster, allowing researchers to test and apply new ways to develop our understanding of the human body and to develop new treatments for diseases. Nowadays, it is possible to explore the use of CRISPR approaches to edit animal genome sequences at will, including mice and zebrafish animal models.
CRISPR is an acronym for Clustered Interspaced Short Palindromic Repeat technique.
The New Scientist described it as 'a way of finding a specific bit of DNA inside a cell. After that, the next step in CRISPR gene editing is usually to alter that piece of DNA'.
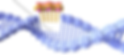
What is also clear is that the efficiency and ease of use of CRISPR - where a faulty gene can simply be switched off and a healthy copy added - has also meant that fewer stages are needed to reach the genetic modifications required by researchers, which in turn reduces the number of animals needed in this process.
CRISPR tools in animal models illustrate why studies like these have been fundamental to develop therapeutic approaches to treat several disorders. These include, numerous research breakthroughs that are beginning to reach the clinical trials stage in humans - and hopefully soon will be available as approved treatments - once they have been tested for their safety and efficacy using animals. Here’s some of the most notable examples, in a whole range of genetic conditions, from recent years:
Sickle-cell disease (SCD)
SCD affects red blood cells, due to gene mutations affecting the haemoglobin – the main protein found in blood, whose function is to transport oxygen from the lungs to the rest of cells in our body. Sickle-cell anaemia patients also develop abnormal red blood cells (sickle-shaped) that can block capillary blood vessels, producing pain and raising the risk of blood clots, while requiring regular (often weekly) blood transfusions.

Using CRISPR tools, first tested in mice, the mutant gene was substituted with another gene. This led to
a successful clinical trial with a small number of patients. In 2019, a woman called Victoria Gray became one of the first people in the world to be treated for a genetically inherited disease with a CRISPR-based therapy. Current results indicate that about 50% of sickle-cell anaemia patients enrolled in this clinical trial have been cured and do not require blood transfusions any more.
Transthyretin amyloidosis congenital (ATTR)
In this rare disease, there is an abundance of the Transthyretin (TTR) gene and a high accumulation of the TTR protein - this is toxic to muscles and nerve cells and can eventually cause premature death.
Researchers have used CRISPR tools to inactivate the TTR gene and have used mRNA technology and lipid nanoparticles (similar to those used for Covid-19 vaccines produced by Moderna or BioNTech/Pfizer) to deliver CRISPR tools via the vein. This was first tested in mice and then in monkeys. Both experiments were successful, and the researchers began a clinical trial whose first patients enrolled have shown a significant reduction in TTR levels in their blood, thereby controlling the disease.
Leber congenital amaurosis type 10 (LCA10)
LCA10 is one of the commonest forms of adult blindness produced by a progressive degeneration of the retina caused by a mutation in the gene called CEP290. Researchers have used CRISPR tools to remove this mutation in order to stop deterioration of the eye. The strategy was first tested in mouse models of this disease and its safety and effectiveness are now being explored in an ongoing clinical trial.
Duchenne muscular dystrophy
In our lab at CNB-CSIC, we have pioneered the use of CRISPR in mice for a basic research project to investigate the functional role of non-coding genome sequences – meaning the part of our genome that does not code the production of any proteins - which represent as much as 98% of our mammalian genomes.

CRISPR genome editing reagents were soon proposed to be used, for gene therapy studies using mice, to first validate these innovative proposals at the preclinical level. Three laboratories, working independently, demonstrated the feasibility of using CRISPR tools on the dystrophin gene carried by the mdx mutant mice, a well-known animal model to study Duchenne muscular dystrophy (DMD). This disease affects the muscles, leading to muscle wasting that gets progressively worse over time.
The corrected dystrophin gene was shown to partially restore the expression of the affected gene in muscles. Similar successful experiments were also carried out in dogs, which naturally suffer from DMD. At the same time, CRISPR technology has facilitated the generation of a variety of additional animal models of DMD in rats, rabbits and pigs that can be used to overcome some of the limitations of the mdx mouse model.
Severe metabolic diseases
The use of CRISPR reagents to develop gene therapy strategies was also tested, using mice, with various severe metabolic diseases associated with perinatal death, such as hereditary tyrosinemia type I (HT-1), and in the urea cycle disorder enzyme, ornithine transcarbamylase (OTC).
EARA member Charles River Laboratories describes the value of CRISPR-based animal models for improving predictability, safety and efficacy in disease research in this feature. Meanwhile, Jennifer Doudna and Joy Wang, working in Doudna's lab, recently published a review looking back on a decade of the CRISPR technology.
CRISPR and reducing animal numbers
One of the most significant impacts of CRISPR technology is on the 3Rs (replace, reduce, refine) and the way these tools can help to reduce the number of animals needed in genome editing.
Often there are a number of breeding steps required to find the correct mutation for the planned experiments, leading to large numbers of animals that are bred but not used. However, CRISPR approaches can drastically simplify the process of creating additional mutations, on top of existing genetic modifications, present in a given mouse model. For example, if a mouse carries two mutant gene variants (alleles), and a third mutation needs to be added, a standard approach would require the creation of this third mutation in a different animal followed by numerous breeding steps between the existing and newly created mutant mice in order to obtain, individuals carrying the three mutations.
As an alternative approach, CRISPR technology, being powerful and reliable, can be applied directly on embryos prepared from the existing mice carrying the two original mutations. And, CRISPR tools can be microinjected into these embryos to generate the desired third mutation. The resulting mice will be born already carrying the three required mutations, cutting down animal numbers by at least 60%.
Further advances and patient-specific treatments
CRISPR genome editing tools can now be used to reproduce in animal models - normally mice - patient-specific mutations associated with a known human disease. The resulting CRISPR-derived mutant mice can be used to study the cause of the disease, to explore a variety of treatments with existing or new small molecules, or to test gene therapy approaches.
A new generation of CRISPR tools called: the base editors (BE), which are considered to have fewer side problems than standard CRISPR tools, are now being used in mouse models to treat rare metabolic diseases, such as phenylketonuria (PKU).

CRISPR tools have also played an important role in boosting the aims of the International Mouse Phenotyping Consortium (IMPC), to create and share mouse mutant models for every single mouse gene with the biomedical community. Every one of these unique mutant mice can provide essential knowledge to understand the role and function of the corresponding copies of all these mouse genes in the human genome.
CRISPR approaches can also be applied to verify spontaneous mutations, found in laboratory mice, with a disease in humans. Specifically, recently we reproduced a mutation found in Naked mutant mice in the Hoxc13 gene, in wild-type mice. These mice suffered from symptoms, such as alopecia with abnormal nails and a short lifespan. This mutation was found to be the equivalent of a rare hereditary human disease known as Ectodermal Dysplasia-9.
The hope for the future is that CRISPR, as its co-discoverer Jennifer Doudna told the BBC: "In the future that this will be a technology that can actually be used not only to understand disease, but also to cure it."
End
**Jinek M, et al. A programmable dual-RNA-guided DNA endonuclease in adaptive bacterial immunity. Science. 2012 17;337(6096):816-21. doi: 10.1126/science.1225829.